Image may be NSFW.
Clik here to view.
Photonic integrated circuits are coming closer to implementing the basic components needed for quantum information processing based on the recent work by EECS graduate student Nicholas Harris, a member of the Quantum Photonics Laboratory headed by Dirk Englund, Jamieson Career Development Professor and principal investigator in the MIT Research Laboratory of Electronics (RLE). [Image above: Optical image of the photonic integrated circuit that creates, filters, and manipulates photon pairs in an area of approximately 0.4 square millimeters. [Optical image, above, of the photonic integrated circuit that creates, filters, and manipulates photon pairs in an area of approximately 0.4 square millimeters. Courtesy N. Harris, Quantum Photonics Laboratory.]
Their work, which was published in Physical Review X in the December 19, 2014 issue, was conducted in collaboration with researchers from the University of Pavia in Italy, a private research firm in Illinois, and the Ecolé Polytechnique Federalé de Lausanne, Switzerland.
The team demonstrated the generation of quantum entangled photons when a strong pump laser beam is sent through a 500 nanometer-wide waveguide (an optical wire) on a silicon photonic integrated circuit. Entangled photons produce correlations in measurements that cannot be explained by classical physics; in this case, the authors showed entanglement in the timing and energy of the two photons. However, a major problem for such sources had been that the pump laser created such strong background light that the entangled photons were barely visible above the noise and required bulky off-chip filters. In their approach, Harris and collaborators attacked this problem by integrating a series of tunable filters on-chip with the entangled photon source — attenuating the pump by a factor of 10 Billion.
In the device, laser light is coupled into the silicon waveguide and subsequently transferred into a micro-ring resonator where the light is trapped while it undergoes many round-trips. Inside the resonator, the atomic lattice oscillates in response to the high intensity of the trapped laser light, absorbing two laser photons and creating two quantum-correlated ones: correlated in their time of creation and their energy.
Mohammad Hafezi, assistant professor of electrical and computer engineering at the University of Maryland’s Institute for Research in Electronics and Applied Physics investigates quantum properties of light propagation and light-matter interaction for applications in classical and quantum information processing and sensing. Not a member of the Englund team that carried out this work, Hafezi notes, “The work by Harris et al. is a remarkable experiment and it is a very important step towards full integration of all photonic components required for large-scale on-chip quantum information processing.”
This new chip-integrated entangled photon source complements another essential component demonstrated recently by the Englund and Berggren groups: single photon detectors based on superconducting nanowire single photon detectors (SNSPDs) [see the Jan. 9, 2015 MIT News story.] The team is now working on combining both sources and detectors in efficient silicon photonic circuits. “This combination should give us a tremendous improvement in how efficiently we can generate and detect quantum states,” said Professor Englund. “This will greatly improve the probability of algorithms for quantum computing and simulation.”
This work was supported in part under a grant from the Air Force Research Laboratory (AFRL/RITA).
[Image below: Photograph of the setup in which quantum-correlated photons are created and filtered in a first chip (see rightmost inset) and then transferred via an optical fiber link to a second chip for further manipulation (see leftmost inset). Courtesy N. Harris, Quantum Photonics Laboratory.]
Image may be NSFW.
Clik here to view.
Research Themes:
News Image:
Clik here to view.
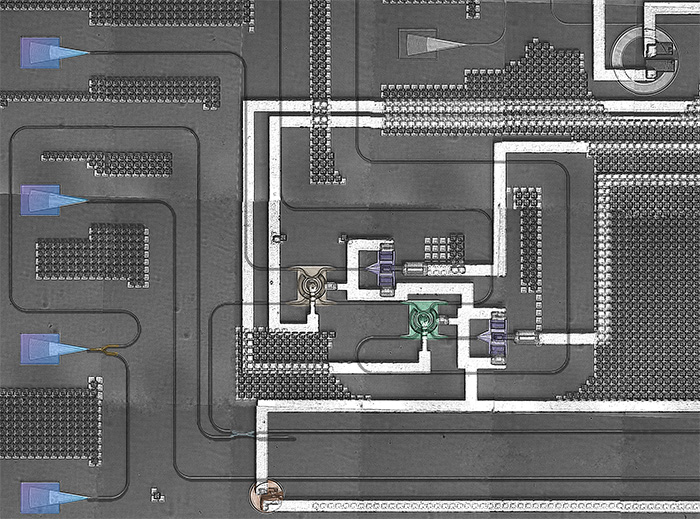